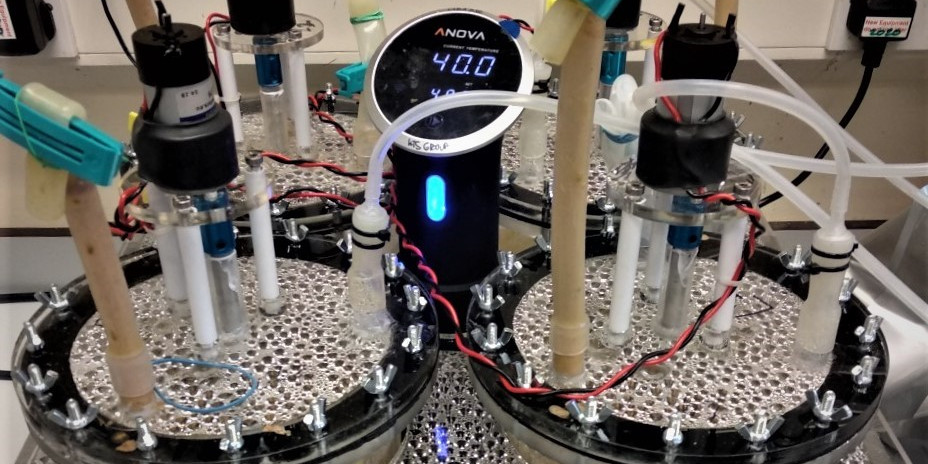
Research to develop anaerobic digestion systems to produce sustainable energy and a safe fertilizer for use in sustainable food production.
Anaerobic digestion (AD) is a naturally occurring microbial process combined with carefully designed engineering to convert human, agricultural and other organic wastes efficiently into biogas for use as a fuel. For instance, commercial AD systems use on–site industrial organic waste to generate heat and power. Other dedicated waste treatment units use locally generated organic wastes, from markets or agriculture, and human waste (from toilet systems) to generate electricity. At the other extreme, AD can use domestic waste to produce biogas as a cooking fuel.
The use of AD to produce biogas is a remarkable example of applied microbiology. In a typical AD system, organic components in the raw materials are converted to organic acids by a set of microbes known as “acidogens”. Then a different set of microbes (“methanogens”) convert the organic acids to a mixture of carbon dioxide and methane. The methane is the key component of biogas. However, there is a problem because too much acid is damaging to the methanogens. This limits the production of methane and the conversion of waste to energy. As a result, in many AD systems much of the organic material remains undigested.
At the end of biogas production AD leaves a by-product (digestate) that is rich in nitrogen, phosphorus and other essential plant nutrients. As a result, digestate has the potential to be a low-cost, locally produced fertilizer for use in sustainable agriculture. However, the use of digestate is limited because, without additional treatments, it can remain contaminated by disease-causing microorganisms (pathogens) present in the original waste material. Contamination can be a problem with food waste, for example obtained from municipal solid waste (“garbage”). It is also a problem with animal manures and especially when the raw material for AD includes human waste, for example from pit toilets or sewage systems.
The additional treatments needed to eliminate pathogens from digestate can include using some of the biogas produced to heat the digestate. This ‘pasteurizes’ the digestate but can also divert a substantial fraction of the biogas produced. Other heat or chemical treatments add significant extra costs.
The combined problems of limited gas production and pathogen contamination of digestates have constrained the adoption and proper implementation of AD systems, especially in developing countries. Improved uptake of AD needs systems that maximize biogas yields AND minimize pathogen load. While there are many different designs for AD systems, no one has produced a system that is optimized to deliver both these goals. This dual optimization is the focus of my research within the GCRF-funded RECIRCULATE project. We are using engineering solutions to separate the acid-producing and methane-producing stages of AD.
This is what we call a “two-stage AD process”. The first stage is known as a plug flow acidogenic reactor or “PFAR”. That simply means that the waste materials enter as a series of batches at one end of the system. Successive batches then flow progressively through the system until they exit at the other end. You can almost think of it like a conveyor belt. We design the PFAR stage to maximise the production of organic acids by acidogenic microbes. The partially digested, acid-rich material from Stage 1 becomes the feedstock for Stage 2. The second stage is referred to as a continuously stirred tank methanogenic reactor (CSTMR). We design the CSTMR stage to optimise the activity of methanogens. This achieves the most efficient conversion of organic acids from Stage 1 to methane, and so maximises overall energy production.
At this point, you may be asking, how does the two-stage process help with reducing pathogen contamination? In theory, high acidity is damaging to pathogenic microbes as well as methanogens. By carefully managing the flow of material through the stage 1 process, we can ensure that organic acids build-up to such high concentrations that they kill the pathogens. Efficient acid production during Stage 1 could be the key step to allowing digestate to be used as a safe fertilizer as well as optimising energy production.
That’s the theory but how does this work out in practice? My research to date has focused on Phase 1. Using lab-scale AD systems I have confirmed that the PFAR approach is very effective at killing a range of pathogens. Even better, the system is more effective at breaking-down the raw materials compared to existing single stage AD. For example, two of the main components of the raw material are cellulose and lignin. In our systems, we find that about 40% of the cellulose and 56% of the lignin is broken down within four weeks. That compares with about 18% cellulose and 16% lignin in typical single stage AD. The next steps will be to ensure that this greater breakdown during Stage 1 is translated in to enhanced biogas yields at the end of the Stage 2 CSTMR process.
The safe and efficient AD processes we are developing will not only produce biogas as a fuel but digestate as fertilizer to boost crop production. To achieve that my engineering research interfaces with RECIRCULATE research across multiple academic disciplines. RECIRCULATE’s interdisciplinary research in the UK, Nigeria, Ghana and beyond aims to deliver solutions for multiple sustainable goals (SDGs). Our sister project ACTUATE will build demonstrator AD systems so that we can get the views of local communities on the best designs for their needs. The ‘headlines’ may be to contribute to Good Health and Wellbeing (SDG3) by improved handling of human and other wastes, which also directly helps SDG6 (Clean Water and Sanitation). However, the RECIRCULATE project does not see those SDGs in isolation. Our new two-stage AD design is a key part of that. Once we have optimized our design then it will allow us to go beyond SDGs 3 and 6 to contribute to SDG7 (Affordable and Clean Energy) and SDG2 (Zero Hunger) as well.
![]() |
Bhushan Gandhi is a graduate researcher working at Lancaster University, UK and is completing a PhD funded by Lancaster University contributing to Work Package 4 (Water for Energy Production) of the RECIRCULATE project. Bhushan has Masters degrees in Environmental Science & Engineering from the Indian Institute of Technology Bombay, Mumbai, and in Biotechnology from M.S. University of Baroda, Vadodara and a BSc in Biotechnology from Pune University, India. Before coming to Lancaster he worked for a pharmaceuticals company in Ahmedabad, India. |
All articles in The FLOW are published under a Creative Commons — Attribution/No derivatives license, for details please read the RECIRCULATE re-publishing guidelines.