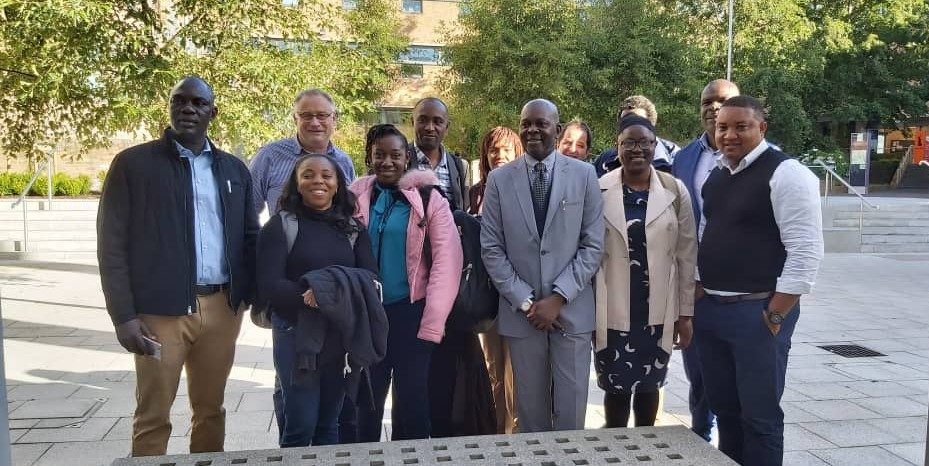
Prof. Alastair Martin shares his personal journey as a chemical engineer into RECIRCULATE, and how the idea of “safe digestate” is coming to life through the project.
Roughly half of the world population- those of us who are lucky enough to live with water sufficiency- probably don’t fully appreciated how completely reliant we all are on water. The other half of us, those living with water scarcity- recognise that clean water is a pressing need, for drinking and cooking, for sanitation and to irrigate crops. Helping communities access clean water and solve the problem of wastewater are powerful motivators for research to find and develop methods to purify and re-use dirty water. As an engineer, the technical challenges of making water and waste safe to re-use are grist to my mill. Even more so if there is also an opportunity to help provide those communities with access to a supply of sustainable energy. The link between these is a process called anaerobic digestion (AD) that produces nutrient-enriched irrigation water and energy from waste and dirty water. That leads to a big question. As a chemical engineer, how have I addressed the challenge of making AD effective and accessible to communities? There isn’t a simple answer. It has been about making connections between seemingly unrelated observations, experience and knowledge, and then testing them through experiments. So let me take you through the stages one step at a time.
I have always been interested in methods of food preservation. One common technique for preserving food is to raise its acidity by the partial fermentation of the food itself. Fufu, garri, sourdough, kindirimo, yoghurt, kefir, nono, sauerkraut, kimchi and salami are all examples. In all these cases fermentation produces lactic acid, which is responsible for the sour taste we enjoy. Even silage used for animal feed is fermented- granted I have not eaten much of that but cows love it! The lactic acid not only prevents the growth of food spoiling organisms but also kills human pathogens- the micro-organisms that make us ill.
While I have always been interested in food preservation, my career has been as a chemical engineer. Working in the wastewater industry, I learned how anaerobic digestion is used to convert the “sludge” separated from sewage into biogas and a nutrient-rich liquid called “digestate”. We gave the digestate liquid to local farmers who used it as a fertiliser on their crops. Inside our anaerobic digesters, micro-organisms converted the raw sludge solids first into liquid, then into acids and finally into the end-products we wanted: biogas and digestate. Effective biogas production needs those three phases, known as “hydrolysis”, “acidogenesis” and “methanogenesis” respectively, to work in harmony. Over time, I helped my employer understand how its anaerobic digesters functioned or perhaps to be more precise, how they did not function. All were reasonably successful as anaerobic digesters making biogas but their success at killing pathogens ranged from, at best, only moderately effective to, at worst, largely useless.
I used my training as a chemical engineer to think about how we might improve pathogen kill in digesters. One basic engineering principle is that a short fat tank with a mixer can achieve things that a long thin pipe cannot and, of course, vice versa. I had learned that the short fat tank with a mixer is just the thing for a digester if you just want to produce biogas but is not much good if you want to kill pathogens. On the other hand, the long thin pipe is excellent for killing pathogens but singularly useless if you want to make biogas. I also learned that short fat tanks were prone to a problem that engineers call “bypassing”, when a proportion of the waste entering the tank sneaks directly to the outlet, arriving there much sooner than it should.
With a team of engineers and technicians I eventually tested every digester that my employer operated which showed me that all suffered to some degree with “bypassing”. As all this information came together, it became apparent that “bypassing” was sufficient to explain all the variability in the killing of pathogens. Underlying this there was a single common coefficient (something chemical engineers call a rate constant) which could explain the speed at which the pathogens were dying. I used this coefficient to work out that the best pathogen-kill I might expect from a “perfect” short fat tank was disappointingly “moderately effective” I could also test whether a long thin pipe could do better at killing pathogens and the answer was an emphatic YES!!! But the test also told me that the harmony between the three phases of digestion that’s needed for biogas production had disappeared completely. Oh dear!
Which comes back to how these seemingly unconnected experiences came together. I started to think back to ideas from food preservation. What if I added the equivalent of the ‘starter culture’ used in many fermented foods? Scientifically the “starter” is called an “inoculum”. With my team at Lancaster University, we did experiments that showed that if we only put a little bit of inoculum in, the digester went acid but didn’t produce much biogas. On the other hand, with lots of inoculum, the digester didn’t go acid but did produce lots of biogas. Drawing a second time on the ideas of food preservation, I realised that that the acidic conditions produced by adding only a small amount of inoculum should be effective at killing pathogens. We did more experiments to test this idea and the results were what we were hoping for. The pathogens died quickly and almost completely, with only 1 in a billion surviving for more than a day. That was great but unfortunately methanogenesis, vital for biogas production, had gone missing.
The final connection combines those insights from food preservation with my training as a chemical engineer. A long-pipe with a small amount of inoculum should be best for killing pathogens. Then we could use the short fat tank that is best for methanogenesis to convert the acidic material into biogas and pathogen free digestate. That’s the theory. Guess what? Yep, we are going to do the experiments, so watch this space!
![]() |
Prof. Alastair Martin is currently the Head of the Chemical engineering discipline at Lancaster University. His research interests are in bio- and thermo-chemical biomass renewable energy, compression rheology and sustainable platform chemicals. From 2014 he developed the Undergraduate programme, achieving accreditation in 2016 with the first MEng graduates and added electrochemical reduction of CO2 to his research interests. He has also patented high efficiency biomass fuelled micro generation process (HiCHP). He is joint leader of the RECIRCULATE Work Package 4 “Water for Energy Production” which, with partners in the UK and west Africa, is investigating the application of anaerobic digestion to the treatment of wastewater and waste for the production of renewable energy |
All articles in The FLOW are published under a Creative Commons — Attribution/No derivatives license, for details please read the RECIRCULATE re-publishing guidelines.